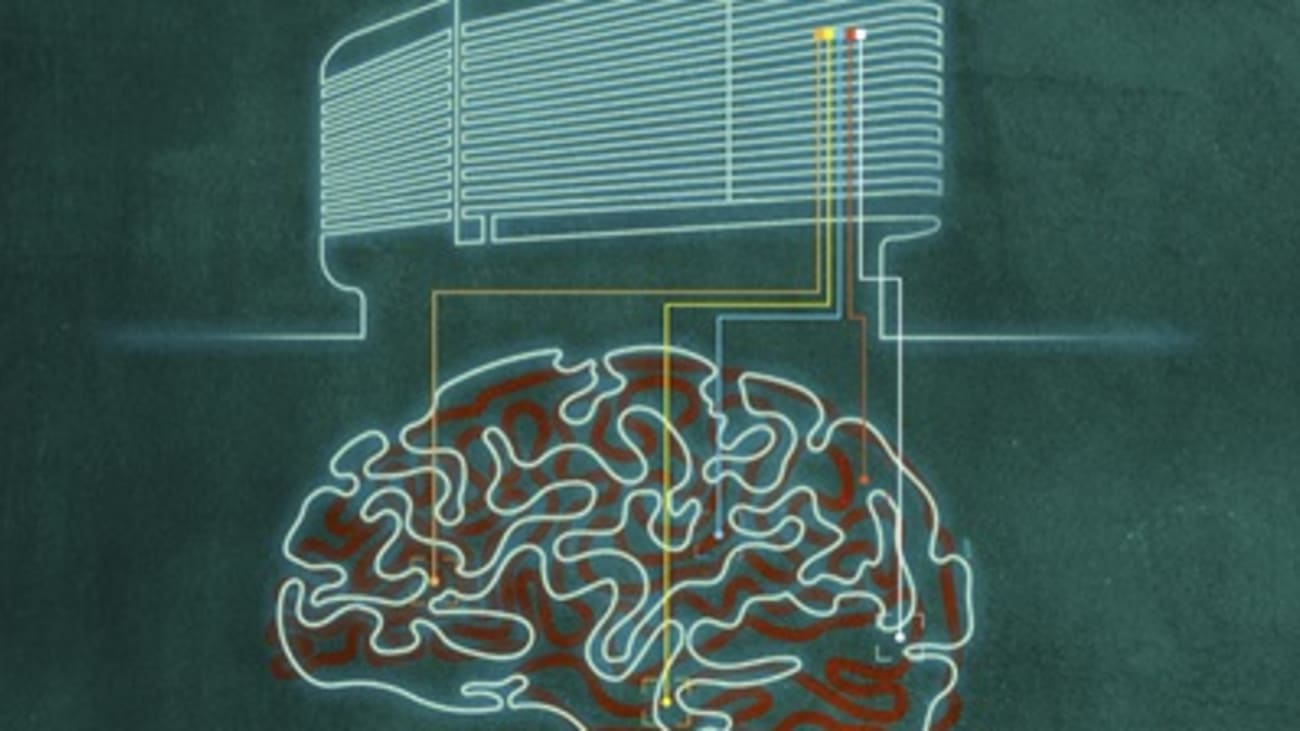
Advanced treatment for epilepsy can offer a unique look at the human mind from inside. By bringing clinical care and neuroscience research closer together, Penn Medicine’s newest inpatient facility will help to forge fundamental neuroscience discoveries and new
neurotechnologies faster and better than ever.
Luke Debevec’s first epileptic seizure came when he was 32. He’d been working on a vacation day — that was typical for him, a young attorney on the cusp of making partner atan international law firm. Then something happened — a storm in his brain. His colleagues found him out cold on the floor of his office.
Four years and countless seizures later, he would find himself sitting in a hospital room at the Hospital of the University of Pennsylvania (HUP). He was plugged into the wall. Multiple electrodes nestled deep into his brain, wires extruding from tiny holes in his head.
He’d tried medication after medication, but nothing had been able to control his epilepsy. Now, doctors were examining activity from these electrodes to show where Debevec’s seizures were coming from in order to plan for surgery that might cure him. But he had also volunteered to let Penn scientists use his wired-up brain as a rare and precious experimental resource.
Neuroscience researchers at Penn depend on patients like Debevec, whose treatment regimen offers a unique opportunity to look at the human mind from the inside.
“Typically patients are just fascinated by being part of the scientific process and being able to contribute to our knowledge of how the brain works,” said Kathryn Davis, MD, MSTR’11, an assistant professor of Neurology at the Perelman School of Medicine and the medical director of the Epilepsy Surgical Program.
“These patients are changing the lives of people in the future by donating their data,” said Timothy H. Lucas, II, MD, PhD, MHCI’19, an associate professor of Neurosurgery and co-director of the Translational Neuromodulation Lab. “And there’s no other way to get that data.”
The studies these patients make possible can sound like science fiction, from defining the fundamentals of consciousness to restoring vision through signals sent directly to the brain.
Soon, research facilities at Penn Medicine’s new Pavilion will bring this collaboration between research and patient care closer together — with a goal to turbocharge these discoveries with new technologies.
Brain Teasers: Core Questions of Consciousness
Alex Proekt, MD, PhD, an assistant professor of Anesthesiology and Critical Care, is collaborating with Tim Lucas, Brian Litt, and Kathryn Davis, on a grant with Max B. Kelz, MD, PhD to better understand consciousness.
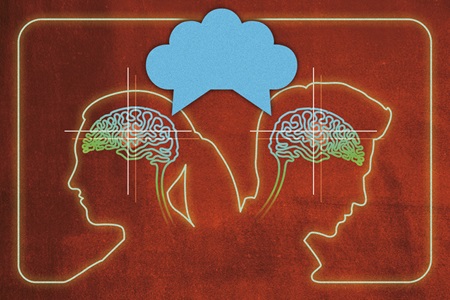
In its broadest sense we are attempting to understand more about how the human brain processes the world around each of us.
We are using anesthetic drugs to initially decode and ultimately predict when the brain is able to perceive its surroundings and when it dives into the unconsciousness of anesthesia. We are similarly giving sensory stimuli to patients to determine in the absence of anesthetics drugs, when the human brain will accurately process sensory stimuli during wakefulness as opposed to losing consciousness during states of natural sleep. This question is also the subject of our studies in basic science.
[We hope] to better understand how neural activity changes from the waking to the anesthetized state and the critical events that must occur to enable individuals to exit the abyss of general anesthesia and recover full cognitive capacity.
— Max B. Kelz, MD, PhD, Professor of Anesthesiology & Critical Care
In the Pavilion, our work will be trying to understand human consciousness. We all have thoughts, feelings, and emotions that happen within our brain that no one else can hear — those things define us and our souls. Our brains are receiving all kinds of sensory information 24/7 and remarkably, our consciousness can filter out the vast majority of this and focus on just on the things it wants to focus on. If you’re sitting in an office chair, you could be sitting for eight hours and not feel your butt even though you’ve been sitting on it all day — the brain has mechanisms to filter out exogenous information. One of our questions is, how does the human brain do that?
— Timothy H. Lucas, MD, PhD, MHCI, Associate Professor of Neurosurgery
Trading a Cart for a Cutting-Edge Research Center
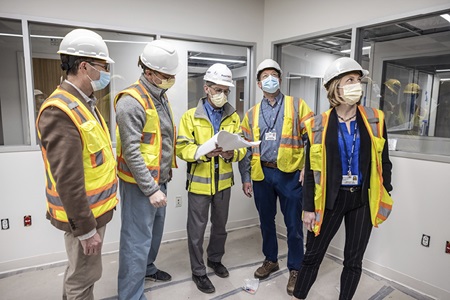
The new Epilepsy Monitoring Unit (EMU) and Human Neurophysiology Research Laboratory will be comprised of 12 patient rooms — doubling the capacity of the current EMU that Davis directs at HUP today — alongside a state-of-the art clinical control room to monitor them. But it will also boast a dedicated lab space for investigators working with patients, with its own suite of technology dedicated to neuromonitoring.
While neuroscientists previously had to trundle carts loaded with equipment from research labs to patient bedsides, the new center will bring investigators from many different labs to work directly with patients in a permanent setting, said Daniel Yoshor, MD, who began as chair of Neurosurgery at the Perelman School of Medicine in the summer of 2020. “There’s limits to what you can do with a cart,” Yoshor said.
The potential for new research will transcend those limits with the opening of the Pavilion, the new 17-story, 1.5-million-square-foot inpatient building on HUP’s campus, this fall. In this location, all of the services patients with neurological illnesses may need will be co-located, including neurology, neurosurgery, neuro-critical care, and step down unit — making it possible for a patient’s clinical care journey to proceed seamlessly as their needs change. This co-location combined with technologies such as an advanced MRI on the unit and integration with data science capabilities to inform neuro-critical care, will make Penn Medicine’s services in these specialties unique in the region.
“These are some of the most technologically intensive areas of medicine,” said Frances Jensen, MD, chair of Neurology. “We are delighted to be moving into a 21st century facility to practice 21st century neuroscience. And with so many different subspecialties in our department, it allows us to do a lot of interdisciplinary work with great partners including neurosurgery and radiology.”
Yoshor first suggested an integrated neuroscience research and clinical center during talks to recruit him from Baylor College of Medicine. He was meeting with University of Pennsylvania Health System CEO Kevin Mahoney, who was telling him about the expanded EMU. Yoshor asked if the EMU could include a dedicated space for scientists to work alongside clinicians.
“What’s so impressive about Penn is that in many institutions the hospital is separate from research, and here it is remarkably integrated,” Yoshor said. Could that integration become even more advanced? Mahoney’s answer was an enthusiastic yes.
“Before, it was going to be a beautiful state-of-art epilepsy monitoring unit, as good as any in the U.S. — but we asked the team and they agreed to add on additional space, dedicated for research,” Yoshor said.
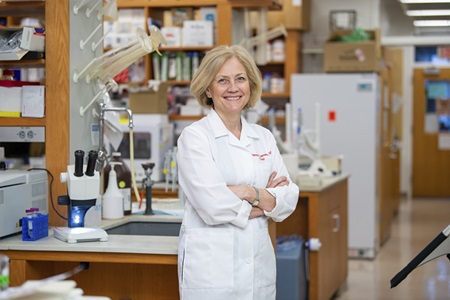
“The neuroscience field is growing exponentially with translation from basic science into clinical implementation,” Jensen added. “We’re seeing a massive uptick in clinical trials that happen at point of care, so, broadly, integration within our new, advanced facility will allow us to expand our offerings to our inpatient population with new biomarker trials, state-of-the-art therapies, and first-in-human therapeutic trials.”
In addition to providing Penn scientists access to the best commercially available equipment, the research space will also function as a testing ground for them to develop their own novel medical devices known as brain-computer interfaces. Using the power of modern computer technology, these permanent brain implants have the potential to head off seizures in epilepsy patients, restore visual function to the blind, and even cure psychiatric disorders.
“It’s basically allowing our EMU not just to be at the leading edge of what’s out there already, but also positioning us to be creating new leading-edge technologies,” Yoshor said.
A key part of the research involves collaboration between clinicians and engineers to develop new types of electrodes that allow for long-term recording and stimulation of the human brain with high precision. This work is being led by Brian Litt, MD, a professor of Neurology and Bioengineering and director of the Penn Epilepsy Center as well as the Center for Neuroengineering and Therapeutics (CNT).
“A tremendous strength of Penn is that our clinicians work side-by-side with great engineers who are experts in computer science and material science, so that clinicians are not just poorly educated users of other people’s technology. We not only avoid all sorts of pitfalls, we build great new technologies together.”
The new research unit “will be one of the very best places in the country or frankly, in the world, to do this kind of science,” Litt added. “I don’t think I’m exaggerating.”
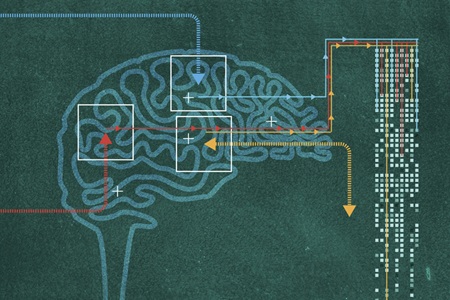
Monitoring the Mind from Inside of It
One auspicious day in 1934, Wilder Penfield bent over the open skull of an epileptic patient. The patient’s brain was open to the air, but the man was awake, under local anesthetic, and able to carry on a conversation even as Penfield, a neurological pioneer, poked at different bits of his gray matter with a small electrical stimulator.
Penfield was hunting for the source of his patient’s seizures, but he found much more.
By stimulating different parts of the brain and watching the patient’s behavior, he could form a rough map of how different parts of the brain work. When the patient’s speech dissolved into gibberish, he knew he’d interrupted part of the brain that processes language. When the patient saw stars, he knew he’d hit a location associated with sight. Stimulating other areas brought other effects — vivid memories, hallucinations, changes in mood.
In 1937, Penfield teamed up with EEG pioneer Herbert Jasper — who initially drove his EEG machines back and forth from Brown University in Rhode Island to the Montreal Neurological Institute to study Penfield’s patients. It was the beginning of the long history of collaboration between people with epilepsy, neuroengineers, and neuroscientists.
“What we learn in this field of neurology is more important to man and more vast than outer space,” Penfield wrote. Yet despite all he and Jasper discovered, he added: “The secrets of the brain and mind are hidden still. The interrelationship of brain and mind is perhaps something toward which scientists and doctors will always struggle.”
It’s a struggle that continues today. Functional magnetic resonance imaging, or fMRI, is noninvasive, requiring no surgery. However, because it measures brain activity indirectly, it has limited resolution. It shows changes in blood oxygenation, and these occur much slower than do changes in neural activity, seconds instead of milliseconds.
To really study the brain in action, you have to go inside one, usually by implanting metal electrodes into the brain tissue. Many important advances have resulted from examining animal brains and their constituent neurons.
“It’s amazing what neuroscience has done studying mouse brains and monkey brains and brain cells in tissue culture,” Yoshor said. “But at a certain level to really understand how the amazing, miraculous human brain works, you have to study human subjects and that’s hard to do. We don’t often get to study awake, behaving human brains.”
Because the implant procedure is risky, it can only be ethically performed in humans as part of a treatment protocol — and drug-resistant epilepsy is the only condition for which implanting electrodes throughout the brain is approved by the FDA. The protocol allows the clinical team to pinpoint the parts of the brain that generate seizures and head them off, either by removing the malfunctioning tissue (termed “resection”) or short-circuiting the malfunctioning tissue with implantable devices.
After electrode implantation, epilepsy patients typically stay in the EMU for a week or so, stopping their medications and waiting for a seizure to come along so its location and behavior can be recorded. Debevec recalls his EMU stay as a “seizure vacation” — “I’m taking vacation to go hang out at the hospital and have seizures, my least fav thing in the world,” he remembered. “And not just to have seizures, but to have them on purpose!”
Taking part in research experiments made the waiting a little less tedious, he recalls. “I participated in whatever I could,” he said. As he was answering questions or completing tasks on the computer to pass the time, all the while the electrons flowed from the wires in his brain to record the particular activation inside.
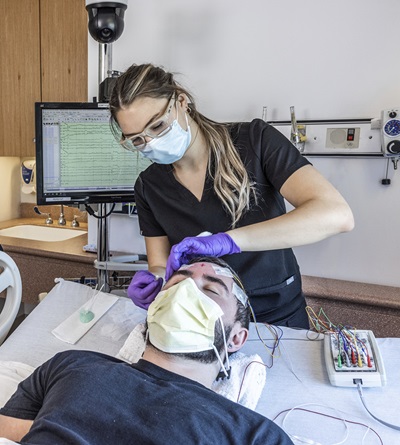
Patients like Debevec are powerful partners in helping scientists study the most advanced and mysterious aspects of human consciousness — such as language, reasoning, or making choices. Take the study Joshua Gold, PhD, a professor of Neuroscience, and neurosurgery resident Ashwin Ramayya, MD’16, PhD’16, have been working on with Timothy Lucas. They’re investigating decision making by looking at the neural activity that governs reaction time.
If you ask someone to do a simple task such as pressing a button when a light flashes, the speed of their reaction will be different every time, even if they see the flash and press the button 100 times in a row. Reaction time variability, or “mental chronometry,” has been a subject of study since the mid-19th century, but it is still not well understood. A computer would complete this task at the same speed every time. Why is a human brain so variable? Why does the decision to press the button sometimes happen quickly, and other times slowly?
“There have been years and years of psychology studies as to what might be happening under the hood, but it has all been guesswork,” Ramayya said. “Amazing guesswork, but there’s a lot of unknowns.”
To observe this phenomenon more directly, the Penn team asked implanted volunteers to complete this task and recorded the neural activity near the various electrodes in their brains. Some of the electrodes were recording groups of neurons involved in the task; others were recording neural circuits that weren’t involved at all.
What they found was surprising. When patients reacted quickly, the neurons involved in the task of pressing the button were firing efficiently — as if someone had cranked a dial from 0 to 11. When they reacted slowly, those same neurons seemed to dither, like someone futzing with a dial to try different volumes before turning it all the way up.
That was to be expected. What they didn’t expect was that the same pattern occurred in neurons that weren’t involved in the task at all. “It was widespread,” Ramayya said. If one group of neurons showed the dithering behavior, others did too.
“The brain is not just a bunch of independent parts,” Ramayya said. “It’s like looking at body of water — it’s not one corner of the water that’s turbulent, all of it is.” The reason humans take a different amount of time to complete the same task might be because the efficiency of information spread in the brain varies from moment to moment — each part influencing the others in an incredibly complex weather pattern. When the weather is calm, ships can carry messages across the sea directly, but when the weather is turbulent, they travel slowly and often take long detours. “Given the data, it’s safe to think that reaction time variability is a brain-wide process,” Ramayya said.
It’s a fascinating observation that couldn’t have been possible without epileptic volunteers.
But for researchers like Yoshor, the goal is not just to observe brain activity, it is to hack it, building a two-way link between the brain and computing devices that can help people recover lost abilities.
Brain Teasers: How an Odorous Molecule Connects to the Sensation of Smelling
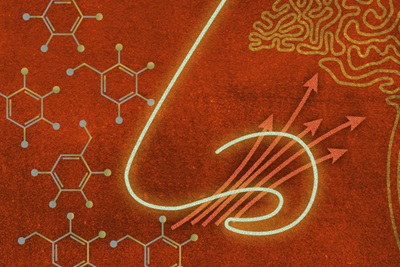
Our research focuses on how odors at the nose are transformed into olfactory signals in the human brain. We use a multidisciplinary set of tools, including task-based functional MRI, intracranial EEG recordings in patients with medically resistant seizures, and anatomical and cellular analysis of the human olfactory system.
The new Pavilion will be instrumental in helping us advance the pace, caliber, and breadth of our intracranial EEG research in epilepsy patients. I expect these amplified resources will place the Perelman School of Medicine as the top U.S. academic medical center pushing the cutting edge of this type of research.
— Jay Gottfried, MD, PhD, Arthur H. Rubenstein Penn Integrates Knowledge Professor of Neurology and Psychology
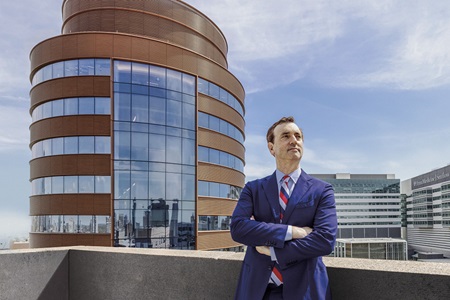
Engineering Sight, Sound, and Touch
Yoshor’s research specialty is visual processing, specifically, creating a brain implant that will allow blind people to see.
For decades, scientists have dreamed of creating a brain “prosthetic” for blindness. By transmitting information directly to the brain’s visual cortex, it should be possible to bypass eyes that have been damaged by disease or injury. Worldwide, nearly a million people wear a similar device for hearing: the cochlear implant, which delivers an auditory signal to the nerve in the ear that the brain then processes into sound — not something comparable to how we hear naturally, but enough to be useful to many people with severe hearing loss.
Creating an equivalent visual prosthetic is more challenging, in part because we take in much more information from our eyes than we do from our ears (while the optic nerve has more than a million neuronal connections between the eye and brain, the cochlear nerve has only about 50,000).
That said, it is relatively easy to understand how artificial stimulation of the optic nerve or visual cortex could work — it is something anyone can do by rubbing their closed eyes. This stimulation makes one see a bright spot, called a phosphene. The basic idea of a cortical visual prosthetic is to produce many such bright spots, using stimulation of electrodes implanted in the visual cortex.
The difficult step is linking different phosphenes to create an image, rather than just isolated spots. It’s easy to assume that “individual phosphenes are analogous to pixels in a computer display and that they can be easily combined to generate a coherent image,” wrote Michael S. Beauchamp, PhD, in a paper recently published in Cell. But “despite its intuitive appeal, there is little evidence that these assumptions are correct.”
Instead, stimulating more than one part of the cortex at the same time just produces blurry blobs of light.
Beauchamp (who recently joined Penn as a professor and vice chair of Neurosurgery), Yoshor and their colleagues came up with a workaround. Instead of stimulating different parts of the cortex all at once, they found a way to stimulate different parts in sequence — creating the appearance of a moving dot of light that could trace the outline of shapes and letters.
The test subjects — blind volunteers as well as sighted epilepsy patients — were able to reliably identify the shapes outlined this way, as well as tell what direction the dot of light was moving in.
It’s a first step toward a viable visual prosthetic that can “put information directly into the brain,” Yoshor said.
“What’s happening in the neurosciences today is extraordinary — there’s been tremendous advances in the past few years and there’s an avalanche of innovation ahead,” he said. “Our efforts are ambitious, but with the ongoing developments in neuroscience, engineering, and computer technology, it is no longer science fiction.”
While Yoshor and his team investigate vision, other investigators will be using the facilities in the new Pavilion EMU to examine other human senses. Yale Cohen, PhD, a professor of Otorhinolaryngology, Neuroscience, and Bioengineering, is studying auditory processing (how we understand, differentiate and think about sounds) while Jay Gottfried, MD, PhD, a Penn Integrates Knowledge professor of Neurology and Psychology, is doing similar work on the olfactory system.
And several, like Yoshor and Beauchamp, are doing research into brain-computer interfaces. Timothy Lucas is building an implanted device to restore a person’s sense of touch.
“When you have a telephone call, two people have equal measure, it’s two-way communication — the same is true for moving an arm or leg. Motor signals from the brain move to the leg to make it contract, or sensory signals from your fingertips when holding a pen send a signal to the brain on how much pressure to apply and when,” Lucas said. “That second connection is called sensory, and the devices we’re working on tackle that side of the equation.”
Many scientists have been working to develop ways to restore movement to paralyzed limbs, or to let people with paralysis operate robotic prostheses, by sending signals from the brain directly to the limb or to the prosthesis. But those advances overlook the importance of the sense of touch, Lucas believes. And experiments in delivering a sense of touch by way of neural implants depend on gauging the subjective experience of human participants.
“Animal studies do not answer the question of how stimulation feels,” Lucas wrote in a 2017 Neurosurgery article. “To answer these qualitative questions, we need human data.”
Brain Teasers: Modeling How Brains Focus on a Fiddle
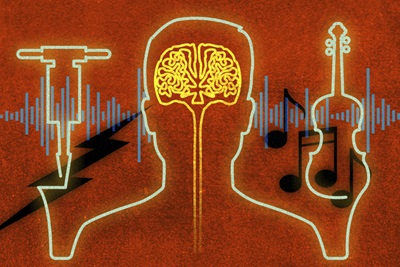
My lab studies how we hear. We study how the brain interprets auditory stimuli as “sounds.” For example, how do we recognize that a fiddle is playing? How do we pay attention to a person’s voice — or ignore it?
We study this by testing behavior and by testing brain function. At the Pavilion, we will be able to conduct more sophisticated hearing studies and be able to collect better and more data. This will facilitate our interactions with the other faculty members in the group so that we can better examine the relationship between neural activity and perception as well as developing computational brain models.
— Yale E. Cohen, PhD, Professor of Otorhinolaryngology, Neuroscience, and Bioengineering
Back to the Bedside
From new forms of computer interface for the able-bodied to radical new ways of augmenting disability, the neuroscience discoveries being made from signals in the brains of epilepsy patients at Penn Medicine could eventually lead to applications in many fields. But Penn’s new Pavilion will also be home to research that will benefit the very people who volunteer their time as test subjects.
At the EMU, Kathryn Davis is already working on ways to improve the outcomes of the patients who come in for surgery.
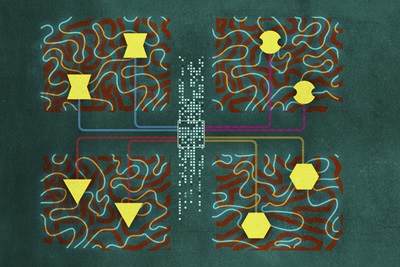
The reason for the electrodes implanted in their brains, after all, is to determine which parts of their brains might be causing their seizures and are good candidates for surgical removal (resection). In some cases, patients are also candidates for permanent brain-stimulating implants, which can prevent seizures by interrupting brain activity with mild electrical pulses, and the electrodes help predict where those implants should go.
Davis’ lab is using machine learning, computational data science and network theory to make this process of prediction more foolproof. One possible route is using computers to perform a virtual resection: before any actual brain tissue is removed, a computer model predicts what the likely outcome would be. Using MRI data to augment the data from implanted electrodes can also provide a clearer picture of how seizures begin.
Concurrently, Brian Litt is working on making seizure-neutralizing implants more functional. He points out that thoughts, feelings, and behavior all have a dramatic impact on human health but implantable devices don’t receive any inputs that track these factors. As he posed the question in a recent grant application, “Coupling peripheral sensors to implants might better infer our actions or thoughts, but wouldn’t it be easier if the devices just asked us?”
He imagines a “new generation of autonomous neurodevices — machines that can question, record, act,” that would interface with users through apps on their phones, warning them if the device registers unusual activity, and learning from the user’s behavior.
For these researchers, this is just a way of giving back to the patient-collaborators on whom their work depends.
“This patient population in particular is incredibly generous with their time, and have a high level of desire to contribute to research even if it’s not going to directly benefit them,” Davis said. “They’ve lived through the frustration of our current knowledge base where there are many holes.”
For Luke Debevec, that was certainly true. Working with Davis, he’d tried many seizure medications; many had terrible side effects, and while some were helpful, none of them stopped his seizures. He was hopeful that surgery would be the answer.
He came through the surgery safely in 2017.
Then he had a seizure. And another one. “I was really depressed,” he recalls. It seemed like he’d done everything he could, and failed.
But today, it has been two years since his last seizure. He’s still on medication, but much less than he had taken before. Where before it had been weeks or months from one seizure to another, now he’s counting in years — an improvement he’s deeply grateful for. “The fact that they can go do brain surgery and I can go back to work and have this interview and reflect about the philosophical points that rise from it — I think that is incredible,” he said. “I am blown away by the whole process.”
More than that, he’s glad he was able to contribute some of his time as a patient to working with the scientists who studied his brain.
“I’m the beneficiary of thousands of years of collective human scientific knowledge,” he said. “At the end of this process, the least I can do is make sure people can continue building on that.”